INTRODUCTION
Durian (Durio zibethinus L.), known as the king of fruits [1], ranks as the most valuable crop in the fruit industry of Thailand, which is ranked the world's number one durian exporter with an export value of USD 1.36 billion in the first quarter of 2023 [2]. Unfortunately, one of major challenges in durian production in Thailand is controlling plant disease, including root and stem rot caused by Phytophthora palmivora, a destructive oomycete plant pathogen [3]. This pathogen can affect different parts of the plant leading to several symptoms, including root and stem rot, leaf and stem blight, trunk canker, and pre- and post-harvest fruit rot [1,4]. Indeed, fruit damage losses of 10-20% have been noted, caused by P. palmivora postharvest fruit rot [1]. Primarily, durian root and stem rot disease management in Thailand relies on recommended fungicide treatment using phenylamides (PAs), quinone outside inhibitors, and carboxylic acid amides [3]. In fact, PAs, such as metalaxyl, are commonly used in the major durian-producing areas, including the Eastern and Southern parts of Thailand. Long-term and extensive usage of fungicide accelerate the risk of fungicide resistance, as evidenced by the discovery of metalaxyl-resistant isolates of P. palmivora in commercial durian orchards in Southern [3] and Eastern [5] Thailand, where metalaxyl has been regularly applied at increasingly higher doses.
Metalaxyl, a systemic fungicide belonging to the phenylamides, was reported to be effective against oomycete pathogens [6]. This fungicide acts as a single site-specific inhibitor toward ribosomal RNA synthesis through interfering with RNA polymerase activity [7], and consequently it affects mycelial growth and zoospore germination [8]. Being a site-specific fungicide, metalaxyl is categorized as high risk regarding resistance development [9]. Soon after the release of the fungicide in 1977 [10], fungicide resistance of oomycete pathogens was reported in the genus Phytophthora of distinct hosts, such as P. infestans on potato [11], P. citricola and P. parasitica on ornamental hosts [12], P. capsic on peppers [13], P. cactorum and P. nicotianae on strawberry [14], and P. palmivora on durian [3]. The molecular basis underlying the metalaxyl resistance mechanism has been dissected in some species of Phytophthora. Sequence variation in gene coding of the large subunit of RNA polymerase I, RPA190, of P. infestans was shown to be associated with metalaxyl resistance [15,16]. Furthermore, the expression of RPA190pc, a homolog gene of P. infestants RPA190, was shown to play role in the regulation of the metalaxyl resistance of P. capsici, by which gene upregulation may be a consequence of the sequestration of metalaxyl, resulting in the unavailability of metalaxyl to target the activity of RNA polymerase activity inside the pathogen [17]. In addition, other proteins besides the large subunit of RNA polymerase I may contribute to metalaxyl resistance. As shown in the study of Vogel et al. [18], a number of single nucleotide polymorphism (SNP) markers associated with mefenoxam (metalaxyl-m) sensitivity of P. capsici anchored to a region of scaffold 62, from where gene encoding a homolog of yeast ribosome synthesis factor Rrp5 was identified among the candidate genes for mefenoxam sensitivity. Indeed, Rrp5 was shown to associate with the formation of 18S and 5.8S rRNA during the yeast ribosome biogenesis process [19]. Conversely, no gene encoding subunit of RNA polymerase I was found from such region. A similar result was also observed in the study by of Marin et al. [8], in which a number of P. cactorum genes with unknown function that had been identified through SNPs were possibly responsible for mefenoxam-resistance. However, no RNA polymerase subunit genes were presented in the list of candidate genes. In recent years, although there have been comprehensive reports of metalaxyl-resistant isolates of P. palmivora associated with durian disease under field conditions, the metalaxyl resistance mechanism in P. palmivora has not been dissected yet. As such, knowledge on the molecular mechanism of metalaxyl resistance in P. palmivora may facilitate monitoring the resistant strains in durian cultivation, leading to an overall design for a proper disease management program.
Based on the above background, the current study was conducted to assess whether RPA1, a homolog gene of P. infestans RPA190, influenced metalaxyl resistance in P. palmivora, the isolate associated the durian disease. The findings obtained should help to establish the basis of the molecular mechanism of P. palmivora resistance to metalaxyl.
MATERIALS AND METHODS
Phytophthora palmivora isolates
In total, 40 isolates of P. palmivora, obtained in 2021 and previously used in the studies of Nianwichai et al. [20] and Thongsri et al. [21], were used as the study samples in the current study. We reconfirmed the species using DNA-barcode based polymerase chain reaction (PCR) with the P. palmivora-specific primers, FM35 and FMPhy-10b [22,23], designed from the CoxII/Internal spacer/CoxI (mitochondrial cytochrome c oxidase subunit II, Internal spacer, and cytochrome c oxidase subunit I) region. Details of the primer sequences are provided in Table 1. All sequences from the CoxII/Internal spacer/CoxI were deposited in the GenBank database under accession numbers OP204950–OP204989 (Supplementary Material Table S1).
In vitro determination of sensitivity to metalaxyl
The 40 isolates of P. palmivora were cultured on half-strength potato dextrose agar (PDA) (HiMedia; Bangalore, India) for 5 days at room temperature. The mycelial plugs (5 mm in diameter) of each isolate were transferred into the center of the Petri dishes (90 mm in diameter) containing PDA amendment with different concentrations of metalaxyl (Table 2) and were incubated at room temperature for 6 days. The experiment was arranged in a completely randomized design with at least 3 replications. Subsequently, the growth diameters of each colony on the culture plate were measured and calculated as the percentage of mycelial growth inhibition using the formula: % growth inhibition=[diameter of colony in control - diameter of treated plate]/diameter of control×100]. Then, the fifty percent effective concentration (EC50) values were calculated using a probit model by linear regressing the probit value corresponding to the percentage of growth inhibition against the logarithm of the fungicide concentration. Next, the EC50 mean values of metalaxyl across repeated experiments of each isolates were calculated, with each P. palmivora isolate clustered into different groups of metalaxyl sensitivity response, as described by Kongtragoon et al. [3], where the isolates with EC50 values<1 mg/L were considered as sensitive (S), isolates with EC50 values of 1 to 100 mg/L were classified as moderately resistant (MR), and isolates with EC50 values greater than 100 mg/L were considered as resistant (R) toward metalaxyl. The dataset of mean EC50 values of metalaxyl for all isolates was used to construct histograms of multimodal datasets from which the frequency distribution of metalaxyl sensitivity was established based on equal proportions of log10 EC50 ranges.
Identification of a homolog gene of Phytophthora infestans RPA190 in Phytophthora palmivora genome
As P. infestans RPA190 is known to be associated with metalaxyl resistance in some studies [15,16], the amino acid sequence of RPA190 from the P. infestans strain T30-4 resistant isolate (accession number: PITG_03855) was used as a reference sequence to search for relative amino acid sequence similarity against the genome of P. palmivora var. palmivora strain sbr112.9, available in the FungiDB database (https:// fungidb.org/fungidb/app) using a BLASTP search. The amino acid sequence that showed the highest similarity to P. palmivora var. palmivora strain sbr112.9, with a maximum score and an E-value close to 0, was further used in this study.
Analysis of DNA and amino acid variance using DNA sequence of Phytophthora palmivora RPA1, the large subunit of RNA polymerase I
Due to the fact that RPA1 (PHPALM_20058) of P. palmivora was identified as the best hit from the above process, we used two primer sets, named as Pal-RPA1ups4-F/Pal-RPA1midR and Pal-RPA1midF/PalRPA1down3-R (Table 1), to obtain the complete DNA sequence covering the RPA1 region of P. palmivora. Indeed, the Pal-RPA1midR and RPA1down3-R primers were designed at the same positions reported by Chen et al. [16] for designed primer sets for DNA amplification of the P. infestans RPA190 region, while the Pal-RPA1ups4-F and Pal-RPA1midF primers were designed from the current study using DNA sequence corresponding to the RPA1 region of P. palmivora var. palmivora strain sbr112.9.
The DNA sequences covering the region of the RPA1 gene from the representative metalaxyl sensitive isolates (CTT2, RKT1, RWT3, TKT3, and TML1), moderately resistant isolates (TML2 and CKKB2), and resistant isolates (CKLB1, CKLL1, TBL1, TBL2, TBL3, and TKL3) of P. palmivora (n=13 in total) were amplified using the two primer sets described above. Genomic DNA of each isolate was subjected to PCR amplification with a total reaction volume of 60 µL, consisting of 500 ng DNA template, 1X PCR Master mix Solution i-StarTaq™ (iNtRON Bio, Seongnam, Korea), 0.5 µM forward primer, and 0.5 µM reverse primer. The PCR reaction was performed under conditions consisting of an initial denaturation at 95℃ for 4 min, followed by 32 cycles of denaturation at 95℃ for 30 s, annealing at 60℃ for 30 s, and extension at 72℃ for 3.30 min, with a final extension at 72℃ for 10 min. The PCR products were electrophoresed through 1% (w/v) agarose gels containing RedSafe™ (iNtRON Bio, Gyeonggi-do, Korea) and visualized under UV light. Then, the PCR products were sequenced with an Illumina BTSeq™ (barcode taq sequencing) by Celemics, Inc. (Seoul, Kore). The sequences of P. palmivora RPA1 were submitted to GenBank under the GenBank accession numbers OQ282371–OQ282380 and PP261190–PP261192..
The heterozygous sites of RPA1 in 10 representative P. palmivora isolates were examined using the Integrative Genomics Viewer (version 2.12.3) [24] with the default parameters. The sequences were translated to amino acids using the ʻtranslate’ tool (https://web.expasy.org/translate/) and SNPs and various amino acids were analyzed using alignment MUSCLE algorithm implemented in ClustalW2 (https://www. ebi.ac.uk/Tools/msa/clustalw2/).
Phytophthora palmivora RPA1 gene expression analysis
The representative isolates of P. palmivora"either resistant (CKLB1, TBL2, and TKL3) or sensitive (RKT1, RWT3, and TML1) to metalaxyl"were selected for total RNA preparation. All above isolates were selected due to their stable growth in the culture media, both in PDA and in potato dextrose broth (PDB; HiMedia, Bangalore, India). All isolates were cultured on half strength PDA for 7 days at room temperature. Subsequently, each isolate was transferred into half strength PDB and cultured for 2 days with 250 rpm shaking. Subsequently, the P. palmivora culture mycelia of each isolate were subjected to a metalaxyl treatment. In detail, metalaxyl was added to the PDB medium at a final concentration based on the EC50 value for each P. palmivora isolate (872.88 mg/L for TBL2, 326.22 mg/L for CKLB1, 103.36 mg/L for TKL3, 0.08 mg/L for RWT3, 0.03 mg/L for TML1, and 0.01 mg/L for RKT1). The metalaxyl treated and non-treated (as control) samples were cultured under the same conditions at room temperature and 250 rpm shaking for 2 h. Subsequently, mycelia from each sample were collected and washed thrice with sterilized water. Total RNA of each sample was extracted using an RNeasy® Plant Mini Kit (Qiagen Ltd., Hilden, Germany), according to the manufacturer’s protocol. The concentration and integrity of the RNA were determined using NanoDrop™ (Thermo Fisher Scientific Inc., Waltham, MA, USA). The first-strand cDNA was prepared using a RevertAid First Strand cDNA Synthesis Kit (Thermo Fisher Scientific Inc., Waltham, MA, USA), following the manufacturer’s protocol and subsequently used as the template for gene expression analysis.
The cDNA samples from each isolate obtained from either the metalaxyl treatment or the non-treatment were quantified using real-time quantitative reverse transcription PCR (qRT-PCR) using the primer set (qRTpal-RPA1F1/qRTpal-RPA1R1) that was designed based on the study by Wang et al. [17]. In addition, the primer set (Lili-ActinHE-F1/Lili-ActinHE-R1) designed from the P. palmivora Actin gene [25] was used as the internal reference. The details of all primer sequences used in this experiment are provided in Table 1. The qRT-PCR was performed in a total reaction volume of 10 µl, consisting of 10 ng cDNA template, 1x HOT FIREPol® EvaGreen® qPCR Mix Plus (Solis BioDyne, Tartu, Estonia), 0.05 µM forward primer and 0.05 µM reverse primer using a Bio-Rad CFX96 q-PCR system (Bio-Rad Corporation, Hercules, CA, USA) with SYBR Green I fluorescent dye detection. Each analysis consisted of two biological replicates and three technical replicates per biological replicate. The relative RPA1 mRNA levels were normalized with the relative expression levels of the internal reference Actin gene using the 2−ΔΔCt method [26]. The RPA1 expression levels were analyzed using one-way ANOVA statistical analysis and Tukey’s test at P<0.05, with the RPA1 expression levels between control non-treated metalaxyl samples and treated metalaxyl samples analyzed using Tukey’s t-test (P<0.05). The correlation between the log10 transformed EC50 values in vitro and the relative expression levels of RPA1 was analyzed using Pearson's correlation coefficient (r).
RESULTS
DISCUSSION
In vitro sensitivity to metalaxyl of Phytophthora palmivora isolates
In vitro sensitivity to metalaxyl assessment among a total of 40 P. palmivora isolates had EC50 values in the range of 0.01-872.88 mg/L (Fig.1, Supplementary Material Table S2), representing a ratio between EC50 of the least and most sensitive isolate tested of 87,288. The large value of this ratio indicated a risk of resistance to metalaxyl in this natural P. palmivora population. According to their metalaxyl sensitivity responses, they were categorized in to 3 groups: sensitive (n=23), moderately resistant (n=11), resistant (n=15). The frequency distribution of the EC50 values formed a multimodal curve (Fig. 2), which confirmed that there were distinct subpopulations based on the metalaxyl sensitivity response levels among all the individuals tested.
Fig. 1
Sensitivity of Phytophthora palmivora (n=40 isolates) to metalaxyl. Metalaxyl sensitivity of each isolate represented as EC50 value. Grouped responses to metalaxyl sensitivity based on Kongtragoul et al. [3], based on isolates with EC50 values<1 mg/L classified as sensitive (S), isolates with EC50 values of 1-100 mg/L classified as moderately resistant (MR), and isolates with EC50 values greater than 100 mg/ L classified as resistant (R) toward metalaxyl.
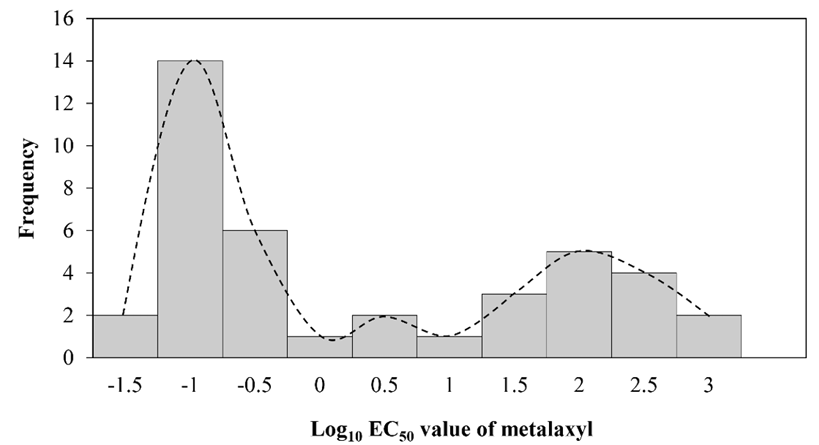
Identification and sequence analysis of Phytophthora palmivora RPA1
BLASTP analysis of the protein sequences of P. infestans RPA190 (PITG_03855) against the P. palmivora sbr112.9 annotated protein database (https://fungidb.org/fungidb/app) resulted in the greatest similarity with P. palmivora DNA-directed RNA polymerase I subunit RPA1 (PHPALM_20058), with a maximum score of 3,482, an E value of 0.0, and 94% identity. The gene structures of P. infestans RPA190 and P. palmivora RPA1 are shown in Fig. 3. RPA1 was 5,745 bps in length (including intron), encoding 1,810 amino acids. DNA sequence analysis of the RPA1 regions among the 13 representative isolates of P. palmivora including the sensitive, moderately resistant, and resistant isolates to metalaxyl revealed no deletion/insertion in this region.
Fig. 3
Gene structures of Phytophthora palmivora RPA1 and of Phytophthora infestans RPA190. Black lines represent intron segments.
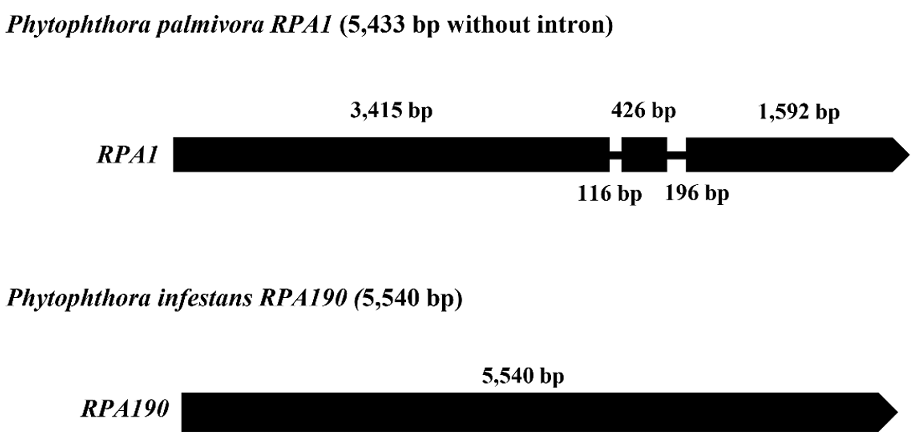
Only one isolate of P. palmivora (TML1) had a single heterozygous synonymous SNP at the A2484T site, which did not produce any change in the amino acid sequence (data not shown). In addition, 100% identical amino acid alignments of RPA1 among the 13 representative isolates were observed (Supplementary Material Fig. S1), inferring that P. palmivora RPA1, a gene homolog of P. infestans RPA190, may not play a role in resistance to metalxyl.
Phytophthora palmivora RPA1 expression analysis
Analysis of the P. palmivora RPA1 gene expression level based on qRT-PCR among the representative metalaxyl-resistant and -sensitive isolates (n=3, each group) displayed the upregulation of the RPA1 gene in some isolates (CKLB1, RKT1, TKL3, and TML1) (Fig. 4). In addition, there was negligible correlation between the relative expression level of RPA1 and the phenotype responsible for the resistance to metalaxyl, with a Pearson’s correlation coefficient of 0.15 (r2=0.02; p=0.78), as shown in Fig. 5. Overall, the results suggested that the P. palmivora RPA1 gene may not play a role in resistance to metalaxyl at a transcriptional control level.
P. palmivora was recognized as the most devastating pathogen of durian plants in Thailand as it causes a variety of symptoms on the plant, including seedling dieback, leaf blight, root rot, trunk cankers, and preharvest and postharvest fruit rot [1] and has been shown to be the predominant causal agent associated with fruit, root, and stem rot of durian cultivated in Southern and Eastern Thailand [3,5,20,21].
Metalaxyl has been recommended to combat durian canker disease caused by P. palmivora for more than the past three decades in Southeast Asia [1]. In oomycetes, resistance to phenylamide fungicides evolves rapidly [27]. The intensive use over a long period fungicidal applications has resulted in the development of P. palmivora isolates that are resistant to metalaxyl as evidenced in other studies, where an increase in
Fig. 4
Relative expression analysis of RPA1 among Phytophthora palmivora representative isolates, sensitive and resistant to metalaxyl (n=3, each group), using real-time quantitative reverse transcription polymerase chain reaction. The relative expression levels of RPA1 in each isolate were normalized to P. palmivora Actin gene. Error bars represent mean±standard deviation (SD) from two independent biological experiments with three technical replicates. Asterisks indicate significant difference at P<0.05 between control samples (non-treated with metalaxyl) and tested samples (treated with metalaxyl) as determined using a t-test. Means followed by different letters are significantly different among representative isolates tested based on Tukey’s test at P<0.05.
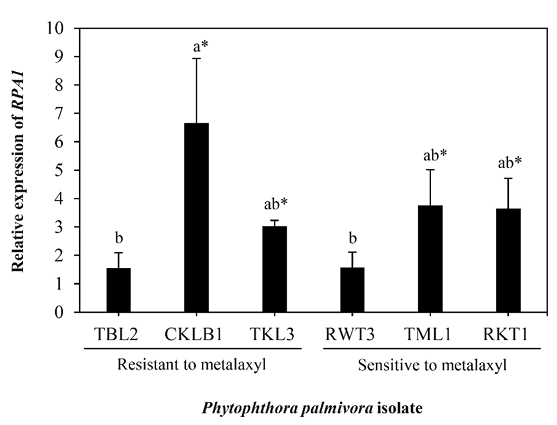
Fig. 5
Pairwise correlation between log10 EC50 value of metalaxyl and relative RPA1 expression level among Phytophthora palmivora representative isolates, resistant (represented as filled triangles) and sensitive (represented as filled circles) to metalaxyl (n=3, each group).
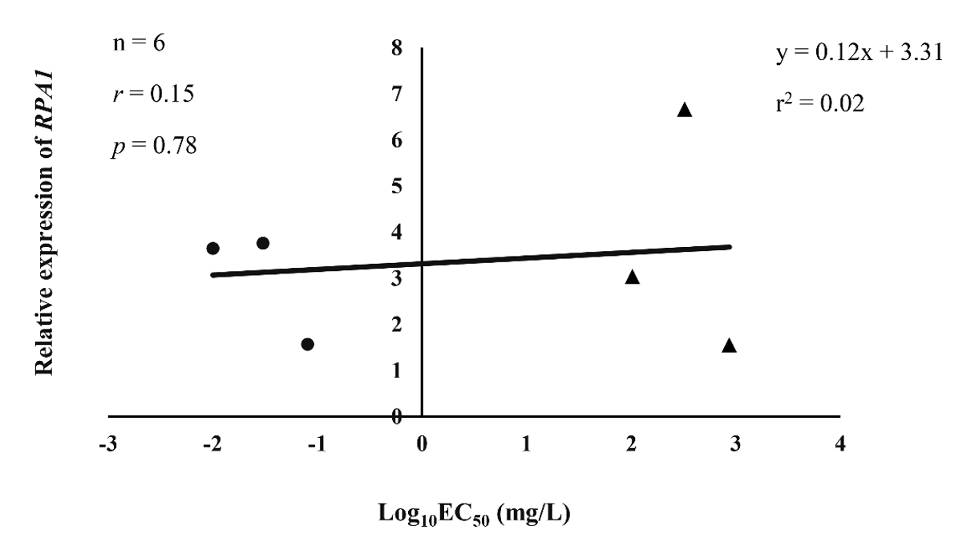
the proportion occurred in metalaxyl-resistant isolates of P. palmivora from 30% in 2014 [28] to 70% in 2017 [3] in the durian orchards in Southern Thailand where metalaxyl has been used regularly for disease management. In addition, Somnuek et al. [5] reported a greater frequency of metalaxyl-resistant isolates of P. palmivora in proportion to sensitive isolates in the P. palmivora population recently obtained from the durian orchards in Thailand, implying that this pathogen is under selection pressure to evade a negative outcome following exposure to the metalaxyl fungicide. Despite the emergence of metalaxyl resistance among the field isolates of P. palmivora, the molecular mechanism of metalaxyl resistance of P. palmivora has not yet been clarified. P. palmivora RPA1 is a homolog gene of P. infestans RPA190 and of P. capsici RPA190-pc, previously known to be associated with metalaxyl resistance [15-17]. Therefore, to establish a f irst perspective on such a mechanism, the current study investigated whether P. palmivora RPA1, a putative gene encoding the DNA-directed RNA polymerase I subunit, had a role in metalaxyl resistance through the analysis of the amino acid sequences decoded from RPA1 and of the RPA1 expression levels between the metalaxyl-sensitive and -resistant isolates of P. palmivora.
Herein the current study successfully obtained representative P. palmivora isolates from both metalaxylsensitive and -resistant subpopulation groups for further examination of the RPA1 gene function associated with metalaxyl resistance. Indeed, in this study, we defined the types of metalaxyl response (sensitive, moderately resistant, or resistant) of each individual based on a range of EC50 values described by Kongtragoul et al. [3]. Although, there was a concern that the data obtained through the same standard protocol may have varied between the different laboratories due to experimental variation [29], there was a considerable range in the variation factor (87,288) between the least-sensitive and the most-sensitive isolates among the current P. palmivora population; furthermore, the metalaxyl multimodal curve could be used to confirm that there was great diversity in metalaxyl sensitivity among the current population. Therefore, the representative P. palmivora isolates selected from either the low or high ranges of EC50 values should be reliable for further assessment of the association between the RPA1 gene and the metalaxyl resistant phenotype.
As mentioned above, the P. palmivora RPA1 gene was a prime focus for the elucidation of a molecular mechanism underlying the metalaxyl resistance in the current study. However, herein we reported that the RPA1 gene may not be involved in metalaxyl resistance in the P. palmivora population derived from diseased durian plants in Thailand. Until now, it was debatable whether the RNA polymerase I subunit (RPA) gene was involved in metalaxyl resistance, since some studies provided evidence supporting such an association [15-17], while other studies failed to demonstrate the RPA gene had such a role [8,18,30]. Although, in the current study there was only a single synonymous heterozygous SNP in a single isolate (TML1) among the whole P. palmivora population, overall, there was no amino acid-based variant of protein encoded by RPA1 among the representative P. palmivora isolates from the distinct groups of the metalaxyl sensitivity phenotype. This finding contradicted the results reported by Randall et al. [15] and Chen et al. [16], where SNP-based variants of RPA190 were found in the P. infestans populations obtained in such studies. Furthermore, SNP T1145A located in the RPA190 gene region could associate with the metalaxyl-resistant phenotype in a majority of the isolates with some particular genotype [15], and multiple SNPs of the AA genotype in RPA190 in relation to the metalaxyl resistant level [16]. No polymorphism in the RPA1 region in the representative P. palmivora isolates in the current study may more likely suggest a non-involvement of the RPA1 gene in the resistance toward metalaxyl. However, it could not be ruled out that RPA1 may be associated with this function in other P. palmivora populations obtained elsewhere, as shown in the study by Randall et al. [15], where the existence of a base T at position 1145 (genotype corresponding to metalaxyl sensitivity) in P. infestans isolates (accounting for 14% of the overall isolates used in that study) was not correlated with the metalaxyl-sensitive phenotype, but demonstrated intermediate sensitivity or resistance toward metalaxyl. Indeed, all isolates showing such a phenomenon were from the same original background genotype. Therefore, additional isolates of P. palmivora obtained from different countries should be included for an association analysis of the RPA1 genotype and metalaxyl resistance function in the future.
In the current study, other evidence to support our postulation on the non-involvement function in metalaxyl resistance of P. palmivora RPA 1 was the lack of a correlation between the RPA1 gene expression and the EC50 levels of the representative isolates. This result was inconsistent with the results presented in the study by Wang et al. [17], where RPA190-pc gene expression participated in the regulation of metalaxyl resistance in P. capsici. Since the representative P. palmivora field isolates showing resistance and sensitive to metalaxyl used in the gene expression analysis were from different origins, they were probably not from the same background genotype. Therefore, it cannot be ruled out that other genes, perhaps existing in some individuals, may affect the metalaxyl resistance function, which may have caused the inconclusive result. In addition, due to the fact that RPA1 expression observed in this study was shown to be constitutively expressed in all the samples tested either with or without the metalaxyl treatment. This may because of its crucial role in cellular protein biogenesis. Since RPA190, a homolog gene of RPA1, encoding of the large subunit of RNA polymerase I, plays role in the synthesis of ribosomal RNA. It is important constituent of the cellular protein synthetic machinery. As shown in the study of Wittekind et al. [31]., conditional expression of RPA190 in Saccharomyces cerevisiae brought about deprivation of RNA polymerase I leading to a decrease of rRNA synthesis and such that caused the growth defect of the yeast.
Notably, metalaxyl affects pathogens through interference of RNA polymerase I template complex DNA [7,32,33], whereas, in the current study, the focus was only on RPA1 (accession number: PHPALM_20058) for the characterization of metalaxyl resistance. Therefore, other predicted proteins of DNA-directed RNA polymerase subunits of P. palmivora should be further characterized for such function.
Although the association of DNA-directed RNA polymerase subunit encoding genes and metalaxyl resistance has been clearly substantiated in the above studies, there was evidence showing that these genes have a negligible function in metalaxyl resistance. The association between the SNP T1145A genotype in RPA190 and metalaxyl resistance was more ambiguous in the study by Matson et al. [30], where such association could not be addressed in some clonal lineages of P. infestans, and co-segregation analysis of the variants derived from the cross of some resistant isolates failed to provide the conclusive result. This result led to their suggestion that RPA190 may not be appropriate for verifying the metalaxyl sensitivity level among the P. infestans population. In the study by Vogel et al. [18], some SNP markers were suggested as candidate genes for mefanoxam resistance associated with mefenoxam sensitivity of P. capsici anchored to a region of scaffold 62 from where a homolog gene of yeast ribosome synthesis factor Rrp5 was identified. Notably, genes encoding the subunit of RNA polymerase I were not found in such a region. Likewise, genome sequencing analysis and SNP variant calling of metalaxyl-resistant and -sensitive P. cactorum isolates in the study by Marin et al. [8] succeeded in identifying potential candidate genes related to metalaxyl resistance, but the RNA polymerase subunit genes were not included.
In conclusion, our study has provided an initial perspective on the molecular mechanism underlying the metalaxyl resistance of P. palmivora, in which the RPA1 gene may not be responsible for the metalaxyl-resistant function in P. palmivora populations associated with root and stem rot of durian in Thailand. A comprehensive perspective on such a mechanism requires further research via a genomewide association study to identify the key proteins affecting metalaxyl sensitivity, which may lead to the development of potential markers for monitoring and controlling P. palmivora metalaxyl-resistant strains and the design of novel inhibitors for future use.