서론
석회암은 지구상에 분포하는 퇴적암 중 20-25%를 차지하고 있으며, 대부분 생물의 생화학적 작용으로 만들어졌다[1]. 우리나라의 석회암 지역은 지리적으로 전국에 걸쳐 나타나고 있으며, 충청북도와 강원도에 집중적으로 분포하고 있다[2]. 석회암 지대의 토양은 pH가 높고, 단립구조(crumb structure)가 발달하여 배수가 잘 되어 다른 토양보다 쉽게 건조해지는 등의 특징을 가지며, 이러한 특성으로 인해 비 석회암 지대의 생태계와는 구조적, 기능적 측면에서 다르게 형성되어 있다[1]. 석회암 지대는 시멘트의 주원료로 사용되는 석회암을 채취하기 위한 광산으로 사용되며 폐광 후의 사면 안정성에 대한 평가가 중요한 문제로 대두되고 있다[3]. 이러한 안정성 문제의 대안 중 석회암 폐광지역의 생태복원이 논의되고 있으며, 해외에서는 생태복원을 위한 연구들이 진행되고 있다[4-6].
석회암지역의 토양에서 자라는 일부 식물들은 백화현상이 유발되어 생장이 저해되기 때문에 생태복원을 위해서는 효율적인 식물생장 방법이 요구된다[7]. 이런 광산들의 생태복원에 있어서 균근균과 공생의 중요성이 잘 알려져 있으며, 균근을 활용한 생태복원을 위해 석회암지대의 목본식물과 공생하는 균근균의 연구가 활발히 진행중이다[8,9]. 석회암지역에서 균근균을 접종하였을 때 식물의 생존에 긍정적인 영향을 준다는 연구결과도 있다[5].
외생균근균(ectomycorrhizal fungi, ECM)은 식물 뿌리에 공생하며 탄소 및 영양소 순환에 중요한 역할을 한다[10]. 식물 뿌리의 외부에 균사가 발달하여 균투(fungal mantle)를 형성하며, 피층 세포들 사이에도 침투하여 하티그망(Hartig net)을 형성함으로써 토양 병원균, 중금속으로부터 숙주식물의 뿌리를 보호하고, 토양 수분 및 여러 양분의 흡수를 높여 식물 생장에 기여한다[11]. ECM의 다양성은 종간의 상호작용, 기후, 숙주식물의 다양성, 토양의 물리‧화학적 특성 등에 의해 영향을 받는다[12-15]. 또한, 토양의 석회화가 ECM의 다양성을 변화시킨다는 연구 결과도 존재한다[16]. 따라서 본 연구에서는 국내 석회암지역에 자생하는 참나무류(Quercus spp.) 뿌리의 ECM 종 다양성과 토양 화학 성분과의 관계를 밝히고자 하였다.
재료 및 방법
토양 및 외생균근균 채집
ECM 시료는 강원도, 충청북도, 경상북도, 경상남도, 전라남도의 총 24개 석회암 지대의 참나무류 뿌리에서 2019년 10월부터 2020년 2월까지 4개월간 수집되었다(Fig. 1). 연구지 내에서 참나무가 우점하는 지역을 선정하여 10 m×10 m의 구획을 설정한 후 무작위로 떡갈나무(Quercus dentata), 갈참나무(Q. aliena), 신갈나무(Q. mongolica), 굴참나무(Q. mongolica)등이 포함된 참나무속(Quercus spp.) 식물의 10개체 뿌리를 채취하였으며, 토양 1 kg과 함께 지퍼백에 넣어 실험실로 운반하였다. 채집한 뿌리는 흐르는 물로 세척 후, petri-dish에 담아 해부현미경 하에서 관찰하면서 뿌리에 형성된 균투를 핀셋을 이용해 무작위로 벗겨 내어 2 mL tube (Eppendorf, Hamburg, Germany)에 옮겼다. 각 연구지별로 채집한 10개의 뿌리에 있는 균투를 무작위로 2 mL tube에 모아 총 24개의 DNA 분자분석을 위한 시료를 만들었다. 또한, 각 연구지별 토양 시료 10개를 100 g씩 모아 24개의 화학성분 분석 시료(1 kg)를 만들었다. 토양 5 g과 증류수 25 mL를 1시간동안 균일하게 섞어준 후 pH meter를 이용하여 토양의 pH를 측정하고, 토양의 전질소함량(%), 유효인산(mg/kg), 치환성 칼슘, 유기물 함량(%) 등의 토양 화학 성분 분석을 의뢰하였다(Cheillab, Seoul, Korea).
외생균근균의 분자생물학적 동정
본 연구에서 외생균근균의 동정에 활용된 분석 방법은 NGS (next generation sequencing)이다. NGS 분석 방법은 시료 내 미생물을 동시에 많은 서열을 분석할 수 있기 때문에 해부현미경으로 확인할 수 없었던 희귀한 분류군을 검출할 수 있어 미생물 군집분석에서 기존의 Sanger sequencing 방법을 대체한다[17, 18]. 하지만, sequence 판독이 200-450 bp로 짧고, 비용이 많이 든다는 단점이 있다[19]. 본 연구에서는 NGS 분석방법 중 빠르고 비교적 긴 염기서열 분석을 통해 정확도를 높여 미생물의 군집분석에 많이 활용되고 있는 Illumina Miseq platform을 통해 외생균근균을 분석하였다[20-22].
각 연구지 별로 2 mL tube에 수집된 24개 시료의 균투는 멸균수를 이용하여 세척한 후 DNeasy Plant mini kit (Qiagen, Hilden, Germany)를 이용하여 genomic DNA를 추출하였다. 이후 ITS3-Mi와 ITS4-Mi 프라이머를 사용하여 Sequencing adapter와 ITS2 지역을 증폭시켰다[23]. PCR의 산물은 전기영동을 통해 ITS영역이 증폭된 밴드를 확인 후 NGS (Illumina miseq method) 분석을 의뢰하였다(ChunLab Inc., Seoul, Korea). NGS 분석결과 종을 동정하고, 이를 토대로 연구지별 참나무류 뿌리의 ECM 상대빈도(relative frequency)를 계산하였다. 이를 토대로 종 다양성 지수(Shannon’s diversity index)를 계산하였고[24], PASW statistic 18 프로그램(IBM SPSS statistics, IL, USA)을 통해 상관분석(correlation analysis)과 회귀분석(linear regression analysis)을 실시하여 ECM 종 다양성과 토양 화학 성분과의 상관 관계를 확인하였다. 또한 principal component analysis (PCA) 방법을 통해 토양 화학성분과 ECM의 관계를 분석하였다.
결과 및 고찰
토양의 화학분석 결과 토양의 전질소함량(TN)은 0.07-0.88의 값이 측정되었으며(Fig. 2A), 유효인산(P)은 5.5-62.8의 값이 측정되었다(Fig. 2B). 치환성 칼슘(Ca)은 0.44-27.23으로(Fig. 2C), 유기물 함량(OM)은 4.24-16.33이 측정되었고(Fig. 2D), 토양 pH는 4.66-7.69로 측정되었다(Fig. 2E). 7개 지역의 토양 pH가 5.5 이하로 측정되었지만, 연구지의 토양 모두 전국 산림토양의 평균 pH 4.30 (국립산림과학원, 2019)보다 높게 측정되었다.
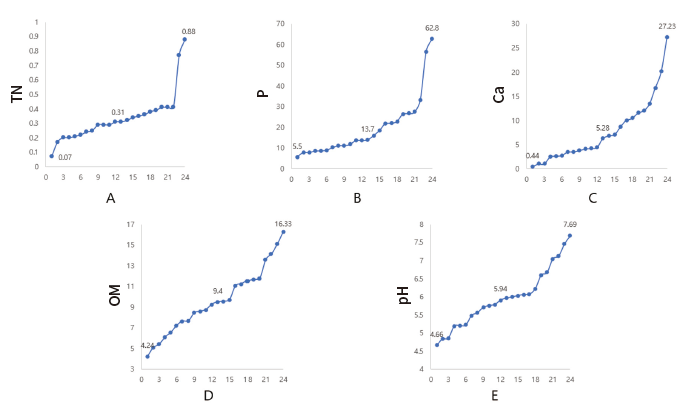
Fig. 2. Soil component analysis of sampling sites. The X-axis represents 24 sample sites, and the Y-axis represents soil components. The values of each graph represent the minimum, median, and maximum. TN (total nitrogen), P (phosphate concentration), Ca (replaceable carsium), OM (organic matter), pH (soil pH).
NGS 분석 결과 석회암 지대 참나무류 뿌리에서 총 1,161,214 reads를 얻었으며, 97% cutoff 조건으로 필터링한 결과, 9,293 mOTU (molecular operational taxonomic units)를 얻었다. 외생균근균에 해당하는 염기서열을 따로 분류하여 군집구조 분석에 활용하였으며, 담자균 72%, 자낭균 17.68%, 접합균 10.32%로 분석되었다. NGS분석을 통해 45속의 서로 다른 ECM을 확인하였다. 상대 빈도를 계산한 결과 Cenococcum속이 가장 높은 빈도로 나타났으며, 그 다음은 Suillus, Sebacina 순으로 확인되었다(Fig. 3). Cenococcum, Sabacina, Lactarius, Tomentella속이 높은 상대빈도를 나타내는 것은 알프스 산의 석회암 지대에서 연구된 가문비나무에 공생하는 ECM의 군집에서 높은 빈도를 차지한 결과와 유사하다[9]. 본 연구에서 Tuber, Cortinarius 속이 낮은 상대 빈도를 나타내고 있는 것 또한 알프스산 석회암 지대의 가문비나무 연구와 비슷한 경향을 나타내고 있지만, Inocybe, Russula 속의 상대빈도는 높게 나타나 다른 결과를 보이고 있다[9].
토양의 화학성분과 ECM 다양성 간의 상관분석을 실시한 결과, pH, 치환성 칼슘, 유효인산의 농도가 증가할수록 ECM의 다양성은 감소하는 경향을 보였으며, 전질소함량, 유기물 함량이 증가할수록 ECM의 다양성은 증가하는 경향을 보였으나(Fig. 4) 모두 유의미한 값을 얻지 못하여 추가적인 연구가 필요할 것으로 보인다. 산림의 석회화는 토양의 산도를 감소시키고, pH가 증가함에 따라 식물 뿌리의 ECM 감염율이 감소한다는 연구 결과가 보고된 바 있다[25-26]. 본 연구에서는 치환성 칼슘 농도와 pH의 증가에 따른 특정 종들의 우점에 의해 종 다양성이 감소한 것으로 생각되며, 높은 인산염의 농도는 특정 ECM 종들의 생장을 억제하기 때문에 유효인산의 농도 증가가 ECM의 다양성을 감소시킨 것으로 생각된다[27]. 또한 전질소함량과 유기물함량의 증가에 따라 ECM의 다양성이 증가하는 것은 ECM이 토양의 유기물을 분해하여 유기질소의 흡수를 증가시켜 식물에게 제공하기 때문인 것으로 보여진다[28].
PCA 분석 결과 석회암 지역의 특징인 칼슘 농도, pH와 양의 상관관계를 갖는 종은 Sebacina, Tomentella, Tuber, Densospora, Inocybe, Suillus, Piloderma속에 속하는 종들이며, 유효인산과 양의 상관관계를 갖는 종은 Amanita, Lactarius, Cenococcum속에 속하는 종으로 나타났다. 전질소함량, 유기물 함량과 양의 상관관계를 갖는 종은 Cortinarius, Thelephora, Meliniomyces속에 속하는 종이고, Tricholoma, Hydnum, Astraeus, Russula속에 속하는 종들은 음의 상관관계를 갖는 것으로 나타났다(Fig. 5). Cenococcum geophilum이 토양의 유기물층을 선호한다는 다른 연구 결과들과는 다르게 본 연구에서는 Cenococcum속이 유기물 보다는 토양 인의 농도와 높은 상관관계를 가지는 것으로 확인되었다[29-31]. 일반적으로 Lactarius속은 질소 친화성(nitrophilic) 균주로서 질소함량이 증가하면서 풍부해지는 반면, 본 연구에서는 질소가 아닌 유효인산과 양의 상관관계를 갖는 것으로 나타났다[32]. Tuber속은 유럽과 중국에서 pH가 높은 석회토양에서 많이 발견되며, 석회토양의 높은 pH와 상관관계를 갖는 것으로 알려져 있다[33-35]. Piloderma속은 유기산의 생성으로 근권 토양에서 양이온 교환 용량(Ca2+, Mg2+, K+)을 증가시킴으로써 광물 토양에서 많은 목본식물들과 공생한다[36]. 산림의 석회화가 매끈한 균투를 형성하는 Russula, Lactarius의 상대빈도를 감소시키고[37], Tuber, Piloderma의 상대빈도를 증가시킨다는 선행 연구결과와 비슷하게 본 연구에서도 Russula와 Lactarius는 칼슘, pH와 음의 상관관계를, Tuber, Piloderma는 양의 상관관계를 갖는 것으로 나타났다[38,39]. 또한 석회토양에서 Tomentella속이 풍부하게 나타난다는 선행 연구결과와 마찬가지로 본 연구에서도 석회토양의 특징인 높은 pH, 높은 칼슘농도와 Tomentella속이 양의 상관관계를 갖는 것으로 조사되었다[9]. Inocybe, Tomentella, Sebacina는 pH 증가에 음의 상관관계를 갖는 것으로 연구된 결과와 반대로 본 연구에서는 3종 모두 pH와 양의 상관관계를 갖는 것으로 나타났다[33].
석회화는 ECM의 종 풍부도를 감소시키지는 않지만 종의 감소나 특정 종으로의 대체를 일으킬 수 있다[38]. 석회화가 진행된 환경에서 적응도가 높은 ECM 종들, 특히 본 연구에서 확인된 Sebacina, Tomentella, Tuber, Densospora, Inocybe, Suillus, Piloderma속의 ECM은 석회토양의 특징인 높은 pH환경과 높은 칼슘 농도에서 잘 적응하는 것으로 보여지며, 이러한 종들에 대한 연구는 석회암 지대에서의 생태 복원에 중요한 기초 자료가 될 수 있다. 또한 참나무 외에 ECM과 공생하는 소나무과, 자작나무과 등의 균근에 대한 후속 연구 또한 이루어져야 할 것으로 생각되며, 토양의 화학성분 뿐 아니라 물리성분에 의한 ECM 군집구조의 영향 및 온도에 따른 영향에 대한 추가적인 연구가 필요할 것으로 생각된다.
적요
국내 석회암지대에 서식하는 참나무류의 뿌리에서 외생균근균(ectomycorrhizal fungi, ECM)의 다양성을 분석하였다. 분자분석을 통해 참나무류에 공생하고 있는 45속의 ECM을 확인하였다. ECM의 종 다양성 지수는 pH, 칼슘 농도, 유효인산 농도가 증가할수록 감소하는 경향을 보였고, 전질소 함량과 유기물 함량이 증가할수록 증가하는 경향을 보였다. 군집분석 결과 석회토양의 특징인 pH와 칼슘 농도와 양의 상관관계를 갖는 ECM은 Sebacina, Tomentella, Tuber, Densospora, Inocybe, Suillus, Piloderma속에 속하는 균주들 이였으며, 이들은 토양 안정성이 약화된 석회암지대의 생태복원에 활용될 수 있을 것으로 판단된다.